Oklo
A natural nuclear reactor
A nuclear fission reactor was operating on Earth at least two billion years ago. Mike Follows looks at the evidence it left behind
EXAM LINKS
The terms in bold link to topics in the AQA, Edexcel, OCR, WJEC and CCEA A-level specifications, as well as the IB, Pre-U and SQA exam specifications.
Analysis of uranium ore samples detected unusual abundances of isotopes. Calculations involving radioactive half-lives help to explain how a natural nuclear fission reactor could have accounted for the results.
Many countries use nuclear fission reactors to generate electricity, but you might be shocked to learn that a nuclear fission reactor was operating on Earth at least two billion years before Enrico Fermi and his team constructed what they assumed was the first nuclear fission reactor in 1942. This article is about Oklo, the fossil of a natural nuclear reactor.
Nuclear fission
Nuclear fission happens when a neutron is absorbed by a ‘parent’ nucleus. The impact changes the shape of the nucleus from a sphere to a dumbbell and the two positively charged ends repel each other, so that the nucleus quickly splits into two daughters, along with two or three neutrons, with a release of energy (Figure 1). These neutrons can induce further fission events as part of a chain reaction.
In a nuclear fission reaction, the combined mass of the product particles is slightly less than the mass of the reactants. This mass difference, Δm, is related to energy, E:
where c is the speed of light (3.00 × 108ms−1). The product particles have greater kinetic energy than the reactants — in other words, they are hotter, and E is the energy output of the reaction.
Figure 1 shows fission of the uranum isotope 235U (uranium-235), which becomes 236U when it absorbs a neutron. When 235U undergoes fission, the daughters are typically barium-139 and krypton-94:

All isotopes of uranium have the same number of protons (92) but different numbers of neutrons. Only 235U is fissile,which means it is the only one capable of sustaining a nuclear fission chain reaction. The uranium found on Earth has a mixture of isotopes, with 235U making up only 0.7202%. To produce a sustained chain reaction, the uranium has to be enriched, which means increasing the concentration of 235U, typically to 3% for power production and 20% for a nuclear weapon.
Missing 235U
Samples of uranium are routinely sent for analysis to ensure that no 235U is being illegally diverted to making nuclear weapons. A mass spectrometer separates particles according to their mass, making it ideal for identifying different isotopes. (For more on mass spectrometery, see ‘Dating with carbon-14’ on pages 6–9.)
In 1972, a test in France discovered a shortfall of 235U in a sample. It came from the Franceville uranium ore deposits near Oklo in the southeast of Gabon, a former French colony in West Africa (Figure 2). There was enough missing 235U to make at least half a dozen nuclear weapons with the destructive power of the one dropped on Hiroshima in August 1945. French physicist Francis Perrin worked out what had happened, perhaps because he had remembered a paper that mooted the possibility of natural nuclear reactors.
Nuclear reactor
In order to understand the natural reactors at Oklo, it is useful to recall how an artificial reactor works. Figure 3 shows a PWR (pressurised water reactor), which is one design of nuclear power station. It needs control rods and water, which doubles as both coolant and moderator. Slow neutrons are more likely than fast ones to cause fission in 235U. A moderator slows down fast neutrons and increases the chance of fission. In its role as coolant, water is pumped around the primary (red) circuit where it cools the nuclear fuel and transfers energy to water in the secondary (blue) circuit.
The water in the red circuit is pressurised to 150 times atmospheric pressure so that it can be heated to 330°C, well in excess of its normal boiling point. The water in the blue circuit is at atmospheric pressure. This is where water turns to steam that spins a turbine. The axle of the turbine is connected to an electrical generator, where a coil and magnets move relative to each other, inducing a voltage and generating electricity.
The control rods are often made of boron or cadmium, which absorb neutrons and act as a brake on the fission process. Without coolant or control rods there is a danger that man-made reactors can undergo meltdown, as happened at Chernobyl in 1986 and Fukushima in 2011.
Natural nuclear reactor
In 1956 Japanese-American scientist Paul Kuroda outlined the four necessary conditions for the operation of a natural reactor. Condition 1 is that at least 3% of the uranium is 235U. The natural abundance of 235U is currently too low for most nuclear reactor designs in use. However, the half-life for 235U is about one-sixth that of the most abundant isotope, 238U (Table 1), which means the proportion of 235U increases as we go back in time, as shown in Figure 4 (see Box 1). 235U accounted for a quarter of all the uranium present when the Earth formed about 4.6 billion years ago.
Condition 2 is that the uranium ore contains at least 10% uranium and that the size of the deposit is at least two-thirds of a metre across, which is the average distance that a fissioninducing neutron travels. Smaller deposits risk too many neutrons escaping before they can induce fission in another 235U nucleus. Sixteen natural nuclear fission reactors were discovered at Oklo — 15 clustered together and one about 30km away (the Bangombé reactor). The biggest is 12m long, 18m deep and between 0.2m and 0.5m wide.
How did the uranium end up where it did? The landscape around Oklo was once a river delta. Streams would have eroded the igneous rocks from across the catchment. Uranium, along with other heavy metals such as gold, would have collected in so-called placer deposits — low-energy places along the streams where water did not have enough kinetic energy to carry the metals further downstream.
The uranium would have remained in these placer deposits had it not been for the Great Oxygenation Event, 2.3 billion years ago, when microorganisms called cyanobacteria appeared that produced oxygen as a waste gas from photosynthesis, just as plants do. This oxygen dissolved in the streams and oxidised the uranium so that it became soluble. Uranium oxide in solution was flushed down all the streams but, once it reached the delta, would have met sediments impregnated with petroleum, which reacts with any oxygen. The uranium oxide was reduced (deoxidised) back to insoluble uranium at this abrupt boundary, where it formed veins of uranium ore. As can be seen in Figure 4, there was a half-billion-year ‘window’ of time between this event and the abundance of 235U falling below 3%, when natural nuclear reactors could form spontaneously.
Because the surrounding sandstones and clays were porous, there was plenty of water present to act as a moderator (condition 3), while condition 4 is that there should be no significant levels of boron, lithium or other ‘poisons’ that absorb neutrons and would prevent a natural reactor even starting.

Modelling the reactor
In 2004 Alex Meshik and his team at the Laboratory for Space Sciences, Washington University, USA developed a mathematical model of how the Oklo reactors behaved. In the model, 7 tonnes of 235U undergo fission, and 2 tonnes of 235U are produced in other reactions, giving an overall decrease of 5 tonnes.
The model uses Equation 1 and data from Table 2 to relate energy output to the mass of 235U undergoing fission (Box 2). Fission of 7 tonnes of 235U gives an energy output of 5 × 1017 J over an operating lifetime of 150 000 years, generating an average power output of 100kW. For comparison, 3kW is the power of a typical fan heater, while the output from the Sizewell B PWR power station is about 1200MW.
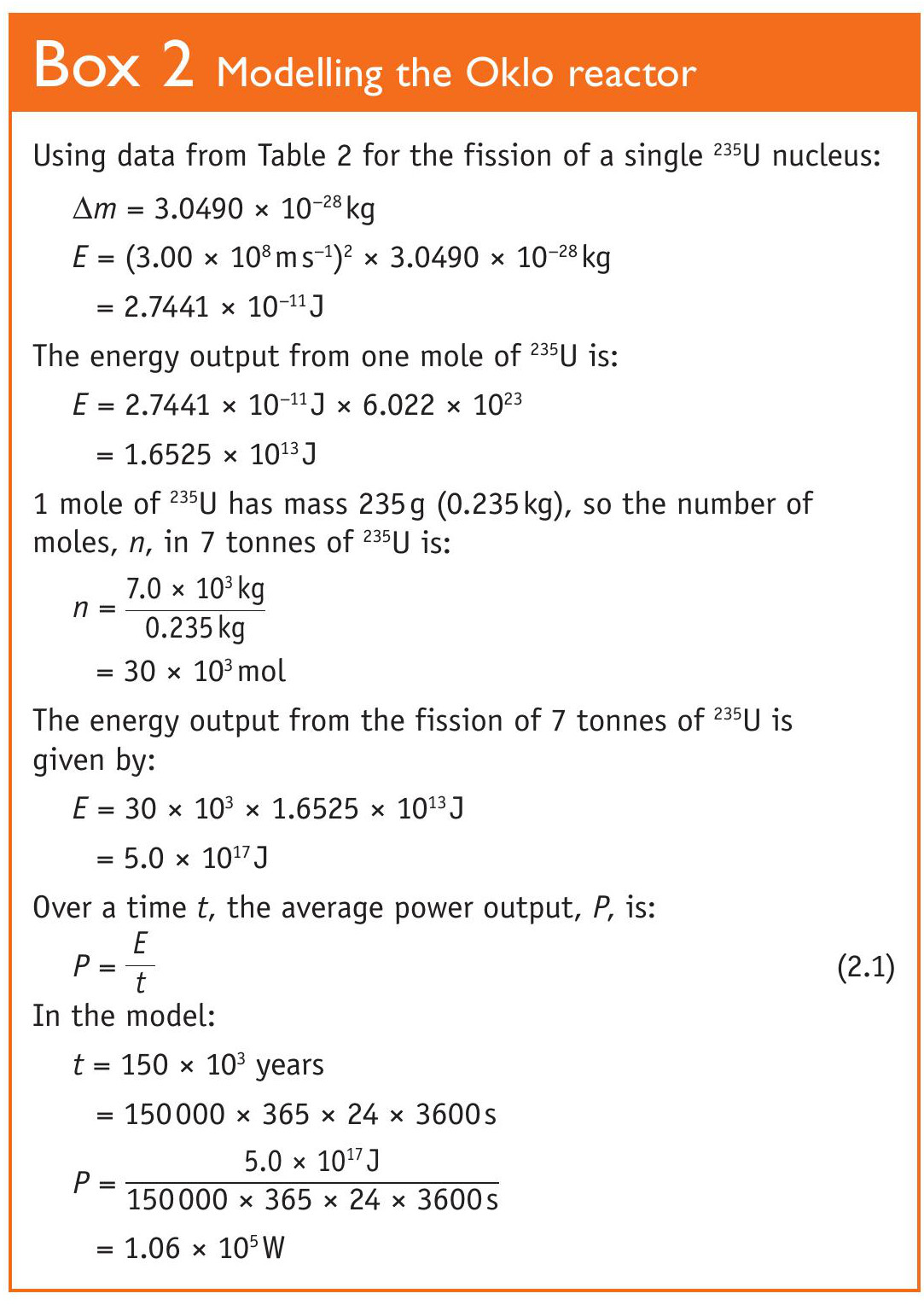
In the model the Oklo reactor would have been ‘switched on’ for half an hour and ‘off’ for at least two and a half hours, which is the typical cycle time of geysers in Yellowstone National Park. This explains why, despite the absence of control rods to control the fission rate, a meltdown or nuclear explosion did not occur. As heating of the reactors increased, the moderator would expand, reducing the density of water molecules that could slow down the neutrons. Fewer neutrons would be moderated and this would reduce the rate of fission. This process, known as a negative temperature coefficient of reactivity, helps to control power in man-made nuclear power stations. The geology of Oklo made it ideal (Figure 5). The underlying granite prevented the water draining away while the porous sandstone allowed water to expand as it heated.
Final comments
A natural nuclear reactor does not need to be pressurised, because it is not trying to maximise efficiency and so can operate at atmospheric pressure. Also, the unique geology of the Oklo deposits meant that the reactors were self-regulating and operated for 150 000 years without a meltdown or explosion. However, a natural reactor could explode and some scientists believe this is how the Moon was created — when a natural nuclear reactor at the core/mantle boundary blew the Earth into two pieces four billion years ago. Oklo data may reveal that what we regard as universal constants may have been different in the past, and suggest that the speed of light may be slowing. But Oklo is not just an academic curiosity — it is teaching scientists about long-term nuclear waste disposal.
PhysicsReviewExtras
Get practice-for-exam questions based on this article at www.hoddereducation.co.uk/physicsreviewextras